New Uses for LAMP (Loop-Mediated Isothermal Amplification)
Abstract
One of the newest methods of nucleic acid amplification, loop-mediated isothermal amplification (LAMP), is a quickly-evolving alternative to PCR that is simpler to perform and has great potential to provide quick point-of-care diagnostics and screening for multiple diseases. This adaptable technique often uses both LAMP-optimized reagents as well as the usual materials required for PCR procedures but without the need for a thermal cycler.
What is LAMP, and Why is it Important?
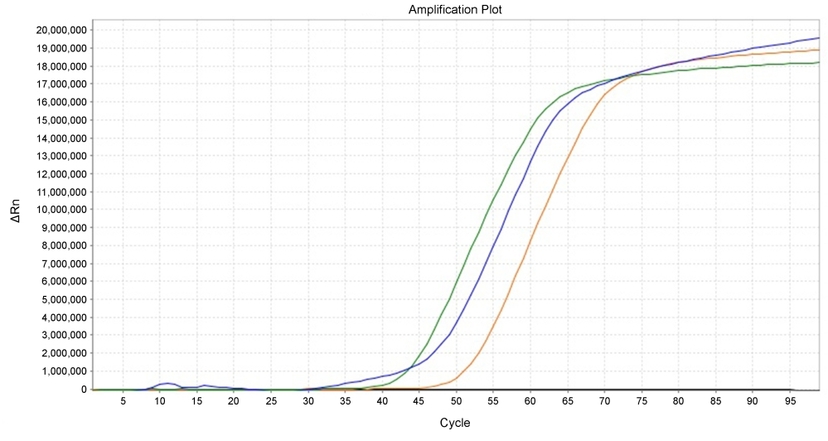
LAMP detection of BRCA1 in HeLa cells. 500 ng (Green), 50 ng (Blue), 5 ng (Orange), and NTC (Black) of gDNA in HeLa cells was used in LAMP reaction with LAMP Green™ fluorescent dye using ABI 7500 qPCR machine.
When compared, PCR has many limiting factors when it comes to field testing that LAMP can overcome. For example, a DNA extraction and purification step is required in PCR, making it difficult to perform at the bedside. LAMP also exhibits less sensitivity to inhibitory substances in biological samples when compared to PCR; this robustness makes LAMP, overall, a less expensive and more rapid technique. Not only is the cost of LAMP considerably lower than other molecular tests, results of a LAMP reaction can be analyzed by the naked eye, without the use of specialized equipment. LAMP experiments also do not require the use of a thermal cycler, raising its potential for use in low-resource settings.
LAMP vs. PCR
Basis Of Differentiation | LAMP | PCR |
Temperature requirements | Is an isothermal process, reaction occurs over a single consistent temperature of 60°C to 65°C throughout | Requires numerous cycles of heating and cooling - reaction occurs over varying temperatures |
Number of primers used | Uses 4 to 6 primers to recognize 6 to 8 distinct regions | Uses two primers to recognize 2 regions |
Reaction time | Rapid reaction, takes less than thirty minutes | Slow reactions, typically takes over one hour |
Sensitivity to sample matrix inhibitors | More tolerant towards sample matrix inhibitors | Highly sensitive to sample matrix inhibitors |
Visual detection | Amenable to visual detection based on certain factors such as turbidity among others | Not amenable to visual detection |
Typical yield | Approx. 10 mg-20 mg | Approx. 0.2 mg |
Equipment needed | Equipment is simpler | Equipment is more complex to accommodate the recurring heating and cooling cycles |
How Does LAMP Work, and What's a Typical Protocol?
.png&w=828&q=100)
Illustrated principle of Loop-Mediated Isothermal Amplification (LAMP), from base target sequence to generation of quantifiable loop complexes produced by targeted primers and polymerization steps. Illustration made using Biorender.
In a standard experiment, LAMP is carried out under isothermal conditions (around 65°C) and usually takes an hour to complete, from start to finish. First, the sample is thermally lysed to release DNA and/or RNA. The primer mix is then added to the reaction, containing FIP, BIP, F3, B3, LF and LB as well as DNA polymerase (typically, Bst DNA polymerase). In the case of reverse transcription-LAMP (RT-LAMP), a heat-stable reverse transcriptase enzyme also must be added to the LAMP mix to derive complementary DNA (cDNA) from RNA present. In conventional 1-step LAMP, all components can be added at once in the same reaction tube. The reaction is allowed to amplify at 65°C for one hour, and then the reaction mix can be denatured through heat and rapidly cooled on ice. The resulting DNA products are formed from repeats of the target sequence, and are extremely long (typically, over 20 kb), connected with single-stranded loop regions in long concatemers. Unfortunately, the amplified products are not usually appropriate for downstream manipulation, but the resulting amplification reaction is substantial enough that multiple modes of detection are possible.
How Can Amplified Products From LAMP be Visualized?
Left: In a 96 well qPCR plate, HeLa cells gDNA (100, 10, 1, 0 ng) were mixed with IsoFAST buffer, BRCA primers mix, 5 mM MgCl2, IsoFAST enzymes, 1 µM DNA dyes (MycoLight™ Green JJ98 (#24000), MycoLight™ Green JJ99 (#24001), Helixyte™ Green (#17592)) and plates were incubated in Applied Biosystems® 7500 FAST Real-Time PCR System at 65 oC (100 cycles for 100 min) for real time detection of fluorescence. No dye negative control (NC): all the component except dyes. Right: Same experiment, verified via gel electrophoresis run in 1% agarose gel, before and after Gelite™ Safe DNA Gel staining. Fluorescence intensity were increased over time in the presence of 1 µM of #24000 and #24001 dye, but not in Helixyte dye. Real time LAMP products were detected in the presence of 1 µM MycoLight dye (#24000,#24001). No amplified products were formed in the presence of 1 uM of Helixyte dye, indicating the Helixyte dye has an inhibitory effect on LAMP reaction. Being able to measure success, failure, and the relative rates of any LAMP reactions that do occur are essential for optimized experimental development.
The detection of DNA from a LAMP reaction has been visualized using one of three methods, including agarose gel electrophoresis. Generally, 5 μL of LAMP products can be clearly visualized under UV light after electrophoresis in appropriate experimental conditions. Gel staining can also be carried out using different intercalating dyes, though regardless of dye used for the visualization, the amount of amplified product is represented by a ladder-like pattern on the gel. The laddered-pattern is due to nonuniformity in the size of the amplified products; sequentially inverted repeats of the target sequence tend to create many inconsistently sized, stemmed and looped DNA.
LAMP reaction products may also be viewed using fluorescence. In this technique, fluorescent dyes like ethidium bromide (EtBr), cyanine dyes (eg., SYBR Green I), or propidium iodide (PI) are also added to the reaction mixture. Amplification analysis can be performed through simple observation without opening the reaction tubes, decreasing the risk of contamination. Normally, a color change indicating the presence of the amplified product can easily be seen, negating the use for any fluorescent-detecting equipment. Moreover, fluorescent dyes normally do not affect the activity of other elements, like enzymes, in the reaction mixture. This method of LAMP analysis includes using turbidity, through ion indicators, as a means of detecting amplified products. This can be accomplished as a major by-product of target amplification is magnesium pyrophosphate, which exhibits as a white precipitate, and can be measured using a turbidimeter or observed by the naked eye.
Historically, visualization methods have been end-based, meaning that determination of the presence of an amplicon is known at the end of an experiment. These techniques are all semi-quantitative, so deciphering the true abundance of an amplicon present in a sample is not always accurate. Further advances and developments in LAMP techniques have led to the creation of real-time technologies that now allow researchers to identify the load of an amplicon in a DNA sample, in real time. Such progress in fluorescent dye and ion indicator methods in particular have allowed for a more accurate quantification of amplified products thereby potentially providing vital information into the viral, parasitic, or even bacterial load on a sample, or more precisely an individual.
How Can LAMP be Used in Diagnostics?
LAMP diagnostic methods have been researched for detecting and diagnosing viral pathogens in humans including for human immunodeficiency virus (HIV), chikungunya virus, mumps, west Nile virus, Zika virus, human papillomavirus, dengue virus, MERS, H5N1 virus, SARS and SARS-CoV-2. LAMP is a significant assay in viral diagnosis because reverse transcription polymerase chain reaction (RT-qPCR) assays are not always equipped to satisfy the current demands of testing large numbers of persons during a viral outbreak or pandemic. Such viruses, if symptoms go untreated, could develop into more serious diseases for example in cervical cancer (from human papillomavirus), AIDS (from HIV), and lung function abnormalities (from MERS and SARS). This means there exists an urgent demand for a rapid, simple and sensitive POC assay that could be used at airports, public transit stations and hospitals, especially in rural areas, to expedite faster detection of viral pathogens and help reduce or prevent spread.
Though LAMP has been used in research to diagnose malaria, LAMP may also be crucial for the diagnosis of other parasitic diseases. The World Health Organisation (WHO) has categorized twenty diseases into a group known as neglected tropical diseases (NTDs), twelve of which are parasitic by nature and are all contagious. These include American trypanosomiasis or Chagas disease, dracunculiasis or Guinea-worm disease, echinococcosis, foodborne trematodiases, human African trypanosomiasis or sleeping sickness, leishmaniasis, lymphatic filariasis or elephantiasis, onchocerciasis or river blindness, schistosomiasis or snail fever, soil-transmitted helminthiases, taeniasis and cysticercosis. Such parasitic diseases affect hundreds of millions of people in developing countries yearly where control is commonly diagnosis-based. As the most affected areas are usually far from laboratories with suitable infrastructure and/or sophisticated equipment, LAMP techniques could therefore significantly help parasite infection control and eradication programs.
Another significant concern related to these diseases is that parasites, once considered endemically stable, are now moving. For example, it has been reported recently that Schistosoma larvae, the cause of schistosomiasis and the second most devastating parasitic disease next to malaria, are infecting people in Europe. The movement of parasites across borders has been linked to, among other things, extreme population growth, urbanization, habitat loss, and seasonal migration. Additionally, climate change and global warming are increasing the dissemination of many vector-transmitted diseases, increasing the likelihood of parasitic outbreaks outside of only rural areas.
LAMP techniques also have the potential to be used in the food industry for testing of bacterial pathogens and fungal contaminants. Numerous outbreaks of bacterial pathogens have be linked to the consumption of food worldwide, and most common bacterial pathogens include Escherichia coli, Staphylococcus aureus, Salmonella, Yersinia enterocolitica, Shigella, Brucella, Campylobacter jejuni, Clostridium, and Cronobacter sakazaki. LAMP may play a significant role in bacterial detection to ensure the safety of food, and to prevent and control food-borne diseases.
Because LAMP is highly efficient, accurate, and sensitive even at low levels of burden, it has the potential to be used to prevent pathogenic transmission through the food chain at every step in the industry, from food production and processing, to marketing and surveillance. LAMP can also be used for bacterial disease diagnosis, as in the detection of meningitis that affects millions yearly, where clinical features alone may not always determine disease presence. In various studies, LAMP has successfully diagnosed meningitis from cerebrospinal fluid samples through detecting the key disease-causing bacteria; Streptococcus pneumoniae, Haemophilus influenzae, and Neisseria meningitidis. Still, the lack of available diagnostic tests in developing countries for bacterial diseases has been highly associated with widespread empiric antibiotic treatment and rising antibiotic resistance. There is an urgent need for effective, bacterial, diagnostic tests, like LAMP, that can help ensure global access to care in both developing and developed countries.
How is LAMP Evolving?
Over the years LAMP technologies continue to improve and integrate with other techniques to create simpler and more quantifiable diagnostic and detection systems. As LAMP continues to evolve, the potential ability and diversity of this technique increases. For example, in one study multiplex LAMP was created that was based on combining two sets of LAMP primers in tandem. A restriction enzyme cleavage site was inserted into the two pairs of each specific primers. This method allowed researchers to distinguish different pathogens simultaneously, using subsequent restriction enzyme analysis (Iseki et al., 2007).
Another variation includes digital LAMP, which was developed using a sample self-digitization chip. Digital LAMP demonstrated an on-chip, loop-mediated, DNA amplification technique that could detect relative changes in template concentration as well as absolute quantification of template numbers (Gansen et al., 2012). LAMP techniques have also been combined with lateral flow assays (LFA), based on DNA hybridization and antigen-antibody reactions. These assays are similar in sensitivity to the conventional agarose gel electrophoresis, but offer a higher level of simplicity, faster testing times, and do not require specialized equipment.
Another system, Lab-on-a-Disc (LoaD) LAMP, was developed that consisted of a fully integrated compact device for the automated detection and quantification of plant pathogens. The construct was a disposable cartridge disc which rotated to generate a centrifugal field. The pressure head pumped the sample from the center to the periphery of the disc, which was then processed, purified, and mixed with LAMP reagents. This instrument also incorporated modules for heating and fluorescent detection (Kinahan et al., 2018).
In another variation of the technique, lyophilized LAMP, all reagents can be combined into a single mixture in a closed amplification and detection system. Not only does this greatly speed up the assay, the user only needs to add water and the sample, or a DNA or RNA template, into the lyophilized mix before incubating. Many lyophilized LAMP kits are commercially available and are commonly associated with portable thermal cyclers that can be connected to a smartphone or tablet for viewing data in real time.
Research has also been done to integrate fluorescence resonance energy transfer (FRET)-based probes with RT-LAMP. These probes were labeled oligonucleotides that operated like a molecular zipper and allowed the quenching strand to displace from a partially complementary fluorescent strand during DNA synthesis. While the targeted double strand region was opened, its fluorophore emitted a fluorescent signal. In this method, pathogenic detection and quantification were performed in real time (Kubota et al., 2011). To date, there are several portable devices based on this technology commercially available.
Resources
Digital Catalog Page:
Related AssayWise Letters:
- Low-cost, ultra-sensitive fluorescence detection of DNA by gel electrophoresis using environmentally benign Gelite™ Safe DNA Gel Stain
- Exploration of SYTO® 9 Variabilities of Labeling Live Bacterial Cells and Analysis of MycoLight™ Fluorophore Alternatives for Live Bacterial Labeling and Viability Assessment
Tools:
Products
Table 1. Dyes for Loop-Mediated Isothermal Amplification (LAMP)
Cat# ▲ ▼ | Product Name ▲ ▼ | Unit Size ▲ ▼ |
17555 | LAMP Green™ *50X DMSO Solution* | 100 µL |
Table 2. Available DNA quantifying reagents for PCR
Product Name ▲ ▼ | Ex (nm) ▲ ▼ | Em (nm) ▲ ▼ | Unit Size ▲ ▼ | Cat No. ▲ ▼ |
Cyber Green™ [Equivalent to SYBR® Green] *20X Aqueous PCR Solution* | 498 | 522 | 5x1 mL tests | 17591 |
Cyber Green™ [Equivalent to SYBR® Green] *10,000X Aqueous PCR Solution* | 498 | 522 | 1 mL | 17592 |
Cyber Green™ Nucleic Acid Gel Stain [Equivalent to SYBR® Green] *10,000X DMSO Solution* | 498 | 522 | 100 µL | 17604 |
Cyber Green™ Nucleic Acid Gel Stain [Equivalent to SYBR® Green] *10,000X DMSO Solution* | 498 | 522 | 1 mL | 17590 |
Cyber Orange™ Nucleic Acid Gel Stain [Equivalent to SYBR® Green] *10,000X DMSO Solution* | 496 | 539 | 1 mL | 17595 |
Gelite™ Green Nucleic Acid Gel Staining Kit | 254 or 300 | Long path green filter | 1 kit | 17589 |
Gelite™ Orange Nucleic Acid Gel Staining Kit | 254 or 300 | Long path green filter | 1 kit | 17594 |
Helixyte™ Green dsDNA Quantifying Reagent *200X DMSO Solution* | 490 | 525 | 1 mL | 17597 |
Helixyte™ Green dsDNA Quantifying Reagent *200X DMSO Solution* | 490 | 525 | 10 mL | 17598 |
Helixyte™ Green Fluorimetric dsDNA Quantitation Kit *Optimized for Broad Dynamic Range* | 490 | 530 | 200 tests | 17645 |
References
Loop-Mediated Isothermal Amplification
Loop-Mediated Isothermal Amplification Methods for Diagnosis of Bacterial Meningitis
Loop-Mediated Isothermal Amplification as Point-of-Care Diagnosis for Neglected Parasitic Infections
Loop-Mediated Isothermal Amplification (LAMP): A Rapid, Sensitive, Specific, and Cost-Effective Point-of-Care Test for Coronaviruses in the Context of COVID-19 Pandemic
Loop-mediated isothermal amplification of DNA
The application of loop-mediated isothermal amplification (LAMP) in food testing for bacterial pathogens and fungal contaminants
Overcoming the global burden of neglected tropical diseases
Loop Mediated Isothermal Amplification: Principles and Applications in Plant Virology