How To Read A Fluorescence Spectrum
by Becky Graves
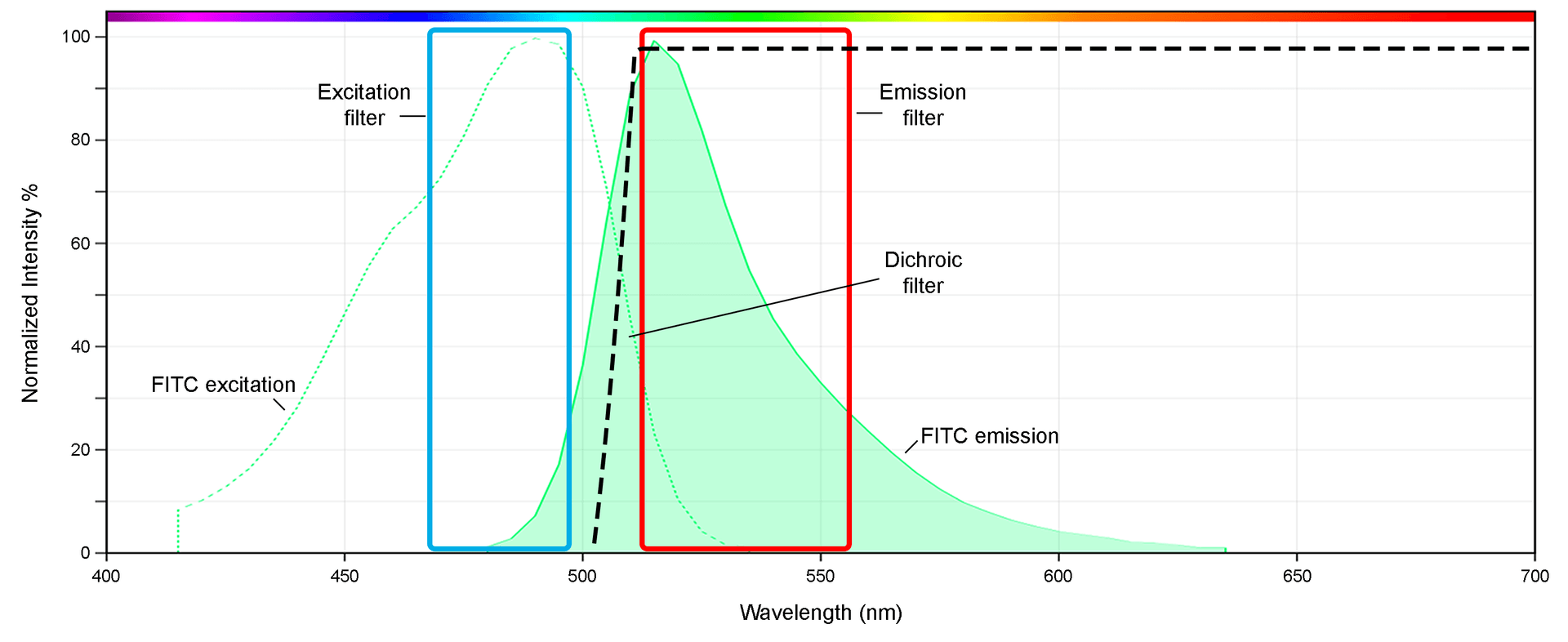
A fluorescence spectrum is an analytical tool that provides researchers and scientists with data based on the way in which a molecule fluoresces. Fluorescence is defined as the three-stage process of excitation, excited lifetime, and emission; while a fluorophore is a chemically diverse molecule that is capable of emitting this light, or fluorescing. Fluorescent molecules have extreme sensitivity and selectivity, and with the use of organic samples, or stains and dyes containing fluorescent components, we can determine various structures and better understand biological processes through analysis and comparison. Fluorescence spectroscopy is commonly used in chemical research and biochemical and medical fields.
The curves of the fluorescence spectrum are often presented in the form of a line graph, depicting the excitation and emission levels of the molecules at a particular wavelength. Reading the spectrum requires an understanding of the excitation and emission stages of a fluorophore, depending on the light of a given wavelength, with respect to the dye used. The x-axis of the spectrum is typically the wavelength (nm), and the y-axis is the frequency, or intensity (%), in which the light emits. This is typically normalized to the highest 'peak'.
All fluorophores reside in a stable, low-energy “ground state” while not fluorescing. It is when the fluorophore begins to absorb the light from an external source that excitation can occur, exciting it to a higher-level electronic state. How much light energy is absorbed will determine the peak of that excited state. In practicality, this translates on the fluorescence spectrum as an excitation peak maximum as well as an emission peak.
In addition, depending on the wavelength and external light source's energy, the excited lifetime of the fluorophore will also vary. The lifetime of the excitation is brief and already beginning to lose energy the moment it begins. The configuration of the fluorophore molecule at this point is semi-stable.
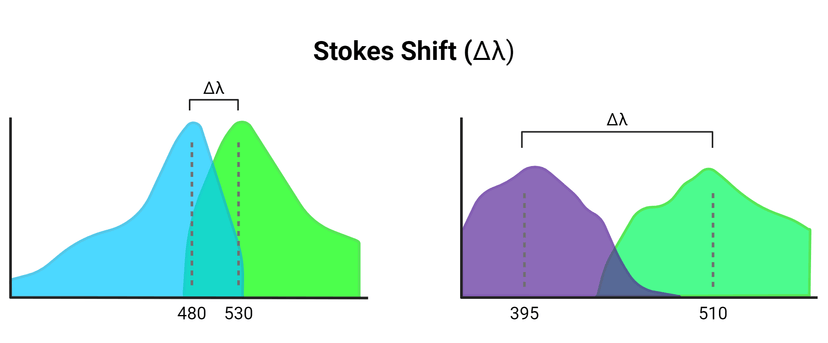
As the fluorophore begins to return to its grounded energy state, a photon of light is emitted. This is the emission stage of fluorescence. As the excess energy becomes released, the wavelength is longer than that of the absorbed light. Therefore, the color of the light absorbed differs from that which is emitted. Because the wavelengths vary between absorption and emission, there will be a shift along the spectrum. This is called the Stokes shift, named after Irish physicist George Gabriel Stokes. Due to the rapid timescale in which the excitation and emission occur, the spectra between the two are nearly identical.
For a given dye used, there is a range of wavelengths in which the light is absorbed, meaning each dye possesses a distinct excitation range. Even within that range, the excitation maximum is achieved as some of the wavelengths are more determined for excitation than others. In the same way that there is a range of excitation, there is a range of emissions. And, in turn, there is an emission maximum that occurs within the range of emission wavelengths. Though the intensity of the light emitted is the same among them, the wavelengths and colors of light are not.
Some fluorophores can be excited and emitted repeatedly, making the fluorescence process a useful tool in allowing for continued studies with more precise molecular specificity and detection. That said, the tolerance of a given fluorophore for multiple excitation events varies, and can lead to a permanent loss of fluorescence behavior known as photobleaching.
It is generally best practice to reduce fluorophore exposure to light so as to reduce the possibility of photobleaching or unnecessary sample degradation. If this is impossible or challenging, antifading reagents and special media are commonly available to improve or sustain fluorescence signal.
In summary, the portions of the fluorescence spectrum include excitation and emission line graphs oriented on an x-axis of wavelength and y-axis of normalized signal intensity. The line curvature will include the 'ground state' which will be low or even zero, then begin to rise when within the relevant range of excitation that correlates to fluorescence. This continues until it reaches its highest point, then begins to lose energy. This visual mountain is the fluorescence curve. The 'ex' and 'em' numbers associated with given fluorophores mark the wavelengths at which the excitation and emission intensities peak, but the entire curve is still relevant to analyze, particularly for researchers looking to minimize fluorescence crosstalk when using two or more dyes in multicolor imaging, or those using spectral flow cytometry, which takes the entire spectral profile of each dye into account when deciphering data.
Fluorescence Spectroscopy: The Basics
Process of Fluorescence
What is a Fluorescence Spectrum?
What is the Stokes Shift?
Fluorescence Spectrum
Original created on March 5, 2024, last updated on March 5, 2024
Tagged under: fluorescence, spectrum, microscopy
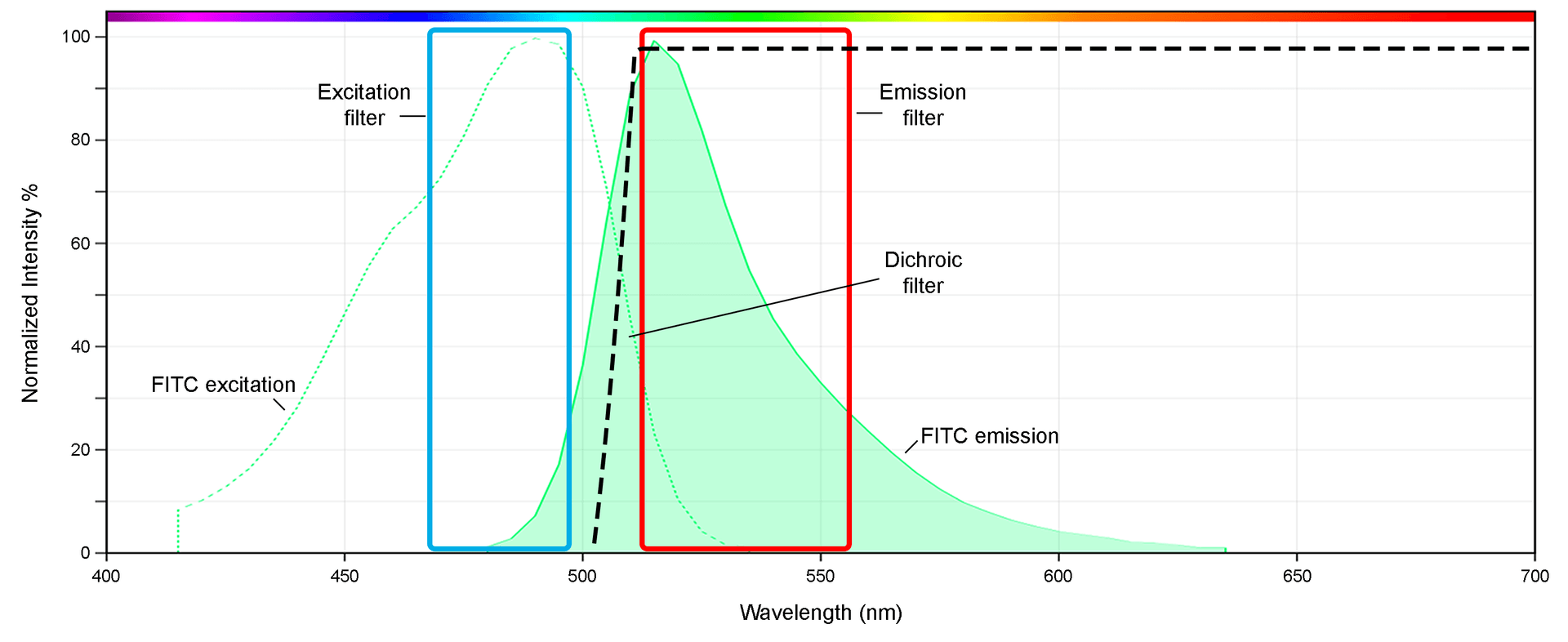
Example of FITC spectrum, including the excitation and emission curves (dashed and solid, respectively) as well as the filter set typically used in microscopy to make best use of the fluorescence signal.
The curves of the fluorescence spectrum are often presented in the form of a line graph, depicting the excitation and emission levels of the molecules at a particular wavelength. Reading the spectrum requires an understanding of the excitation and emission stages of a fluorophore, depending on the light of a given wavelength, with respect to the dye used. The x-axis of the spectrum is typically the wavelength (nm), and the y-axis is the frequency, or intensity (%), in which the light emits. This is typically normalized to the highest 'peak'.
All fluorophores reside in a stable, low-energy “ground state” while not fluorescing. It is when the fluorophore begins to absorb the light from an external source that excitation can occur, exciting it to a higher-level electronic state. How much light energy is absorbed will determine the peak of that excited state. In practicality, this translates on the fluorescence spectrum as an excitation peak maximum as well as an emission peak.
In addition, depending on the wavelength and external light source's energy, the excited lifetime of the fluorophore will also vary. The lifetime of the excitation is brief and already beginning to lose energy the moment it begins. The configuration of the fluorophore molecule at this point is semi-stable.
Common Types of Fluorescence Microscopy: | ||
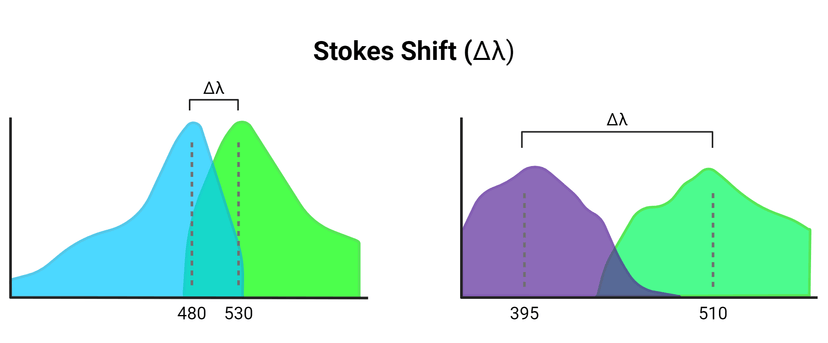
Schematic representation of varying wavelength differences between excitation and emission spectral curves (Stokes Shift) as a consequence of energy loss due to fluorescence activation. Figure made in Biorender.
For a given dye used, there is a range of wavelengths in which the light is absorbed, meaning each dye possesses a distinct excitation range. Even within that range, the excitation maximum is achieved as some of the wavelengths are more determined for excitation than others. In the same way that there is a range of excitation, there is a range of emissions. And, in turn, there is an emission maximum that occurs within the range of emission wavelengths. Though the intensity of the light emitted is the same among them, the wavelengths and colors of light are not.
Some fluorophores can be excited and emitted repeatedly, making the fluorescence process a useful tool in allowing for continued studies with more precise molecular specificity and detection. That said, the tolerance of a given fluorophore for multiple excitation events varies, and can lead to a permanent loss of fluorescence behavior known as photobleaching.
It is generally best practice to reduce fluorophore exposure to light so as to reduce the possibility of photobleaching or unnecessary sample degradation. If this is impossible or challenging, antifading reagents and special media are commonly available to improve or sustain fluorescence signal.
In summary, the portions of the fluorescence spectrum include excitation and emission line graphs oriented on an x-axis of wavelength and y-axis of normalized signal intensity. The line curvature will include the 'ground state' which will be low or even zero, then begin to rise when within the relevant range of excitation that correlates to fluorescence. This continues until it reaches its highest point, then begins to lose energy. This visual mountain is the fluorescence curve. The 'ex' and 'em' numbers associated with given fluorophores mark the wavelengths at which the excitation and emission intensities peak, but the entire curve is still relevant to analyze, particularly for researchers looking to minimize fluorescence crosstalk when using two or more dyes in multicolor imaging, or those using spectral flow cytometry, which takes the entire spectral profile of each dye into account when deciphering data.
Tools: |
References
Fluorescence Spectroscopy: The Basics
Process of Fluorescence
What is a Fluorescence Spectrum?
What is the Stokes Shift?
Fluorescence Spectrum
Original created on March 5, 2024, last updated on March 5, 2024
Tagged under: fluorescence, spectrum, microscopy