Cyclic AMP (cAMP) Signaling
The cyclic adenosine 3'/ 5'-monophosphate (cAMP) signal pathway, is a G-protein mediate cascade of biochemical reactions associated with various hormone specificities and neurotransmitters. cAMP is a cyclic, second messenger, nucleotide that interacts directly with a handful of main targets; protein kinase A (PKA), cyclic nucleotide-gated ion channels (CNGCs), and associated exchange proteins (EPAC). These pieces are linked to a number of vital downstream signal pathways, including metabolism, calcium handling, gene expression, vascular ability, cell proliferation and apoptosis.
Table of Contents
cAMP Regulation
Initiation by ACs
The cAMP signal pathway starts with the downstream activation of Adenylyl cyclase (AC) by the G-⍺ subunit on G-protein-coupled receptors (GPCRs). After activation by GPCRs in the plasma membrane, AC effectively converts adenosine triphosphate ATP into cAMP. Numerous cAMP molecules can be generated in the cytosol, thereby intensifying the outward signal onto target sites. In mammalian cells there are at least 10 known AC isoforms, each with similar architecture, but also with variable enzymatic ability and modulatory domains. Most however are related to Calcium (Ca) channel activity.
Application Notes: |
Termination by PDEs
Phosphodiesters (PDEs) terminate cAMP molecules by compartmentalization and degradation and are critical to the temporal and spatial regulatory dynamics of cAMP signaling. PDEs over the years have shown remarkable complexity, and over 100 different isoforms have been identified to date. Enzymatic differences arise due to the at least 21 different associated encoding genes, alternative splice sites and various transcription initiating points. Though structure may be similar, isotypes are functionally unique.
Table 1. PDE Substrates
Cat# ▲ ▼ | Product Name ▲ ▼ | Unit Size ▲ ▼ |
13602 | FAM-cAMP PDE IV substrate *Green Fluorescence* | 0.5 µmol |
13603 | TAMRA-cAMP PDE IV substrate *Red Fluorescence* | 0.5 µmol |
13604 | FAM-cGMP PDE V substrate *Green Fluorescence* | 0.5 µmol |
13605 | TAMRA-cGMP PDE V substrate *Red Fluorescence* | 0.5 µmol |
cAMP Targets and Subsequent effects
Protein kinase A (PKA)
After cAMP binds to regulation sites on PKA, it may dissociate into 7 different regulatory and catalytic subunits that initiates an ensuing signal cascade by phosphorylation. Downstream effectors of PKA have been linked, including cardiomyocytes, endothelial cells, adipocytes, neurons and more. PKA phosphorylation also influences transcription factors in target genes, nuclear receptors and thyroid stimulating hormones. In the looping nature of the cAMP pathway, PKA as well interacts with EPACs and ion channels in the plasma membrane, further stimulating the flow of calcium, sodium, and potassium.
Datasets: |
Exchange Proteins (EPAC) and GTPases
EPACs are guanine nucleotide exchange factors (GEF) that activate certain GTPases, Rap 1 and 2. These small G-proteins are involved in an array of cellular activities, including adhesion, exocytosis, macrophage rearrangement, cytoskeletal kinetics and restructuring. EPAC 1, after cAMP activation, tethers to the plasma membrane, while EPAC 2 interacts directly with activated G-proteins, namely Ras. EPACs have been shown to play a role in cell migration and proliferation in some types of cancer, making them a potentially ideal target for oncological therapies.
Application Notes: |
Cyclic nucleotide-gated ion channels (CNGCs)
CNGCs are directly influenced by the activity of cyclic nucleotides as nonspecific cation channels. Increased concentrations of cAMP (and cGMP) upregulates activity linked to the Ca signaling pathway, which stimulates calmodulin (CAM) and associated proteins, which reciprocally regulate the activity of AC and PDE. CGNCs function is crucial to maintaining cell homeostasis through regulation of de- and hyperpolarized events. CGNC activity has also shown significance in kidney function, olfactory and photoreceptor response.
Application Notes: |
cAMP and Pathophysiology
Due to the broad range of function and association, cAMP concentration has many effects in regard to immunity in the body. It has been shown that intracellular cAMP levels have negative effects on production of monocytes and granulocytes, thereby decreasing inflammatory effects.
- In natural killer (NK) cells, a cell that suppresses tumor cell growth, cAMP levels regulate cytotoxicity.
- In endritic cells (DCs), cAMP suppresses and promotes pro- and anti-inflammatory mediators, respectively, to regulate T cell immunity.
- In B cells, cAMP stimulates antibody production.
Measuring cAMP
Fluorescence resonance energy transfer (FRET)
FRET technology optically exploits the inner workings and complexities of the cAMP signaling pathway. The biosensors used contain a cAMP binding domain, PKA or an EPAC, sandwiched in between two fluorescent proteins. Even traditional optical microscopes provide the ability to visualize cAMP in real time, quantify concentration levels, and view interactions between GPCRs. Advancing FRET technologies have genetically modified biosensors to target cAMP at specific subunits, thereby providing an even more intuitive map of the signal process. FRET assays can provide readouts of fluctuating cAMP and Ca levels over time, and has recently been developed for high-throughput capabilities.
Table 2. FRET No-Wash For cAMP Assay Kits
Cat# ▲ ▼ | Product Name ▲ ▼ | Unit Size ▲ ▼ | Ex ▲ ▼ | Em ▲ ▼ |
36379 | Screen Quest™ TR-FRET No Wash cAMP Assay Kit | 1 plate | 390 | 650 |
36380 | Screen Quest™ TR-FRET No Wash cAMP Assay Kit | 10 plates | 390 | 650 |
36381 | Screen Quest™ TR-FRET No Wash cAMP Assay Kit | 50 plates | 390 | 650 |
Enzyme linked immuno-assay (ELISA)
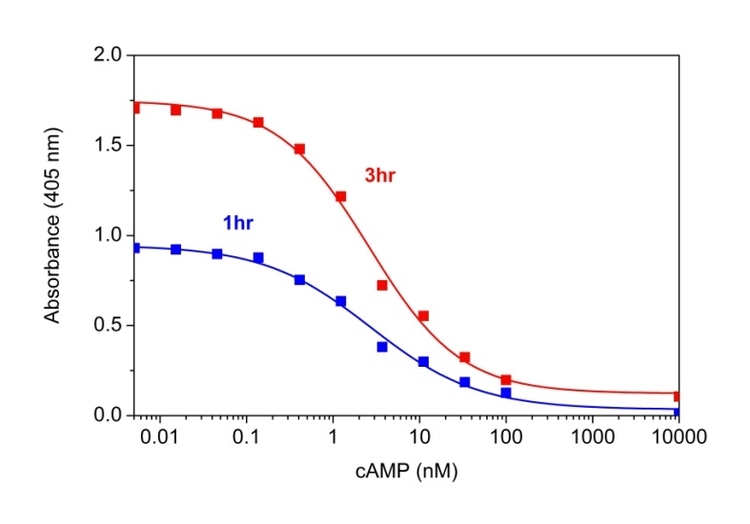
cAMP dose response was measured with Screen Quest™ Colorimetric ELISA cAMP Assay Kit. A: The kit can detect as low as 0.1 nM cAMP in a 100 µL reaction volume at 405nm after incubation with Amplite® Green for 1 hour (blue line) and 3 hours (red line).
Table 3. A Comparison of Available cAMP Assays Using cAMP Standard Curves
Product Name ▲ ▼ | Supplier ▲ ▼ | Description ▲ ▼ | IC50 ▲ ▼ |
Screen Quest™ Colorimetric ELISA cAMP Assay Kit | AAT Bioquest | Direct competitive ELISA without acetylation | 2.6 nM |
cAMP Assay Kit | Abcam | Direct competitive ELISA with acetylation | 90.4 nM |
cAMP Parameter Assay Kit | R&D Systems | Direct competitive ELISA without acetylation | 30.5 nM |
Lance cAMP Assay | Perkin Elmer | Time-resolved FRET with anti-cAMP antibody | 3 nM |
cAMP-Glo Assay | Promega | Luminescence from ATP-coupled, PKA-dependent luciferase reaction | 8.3 nM |
GS Dynamic Kit | Cisbio | Time-resolved FRET with anti-cAMP antibody | 5.6 nM |
Product Ordering Information
Table 4. ELISA For cAMP Assay Kits
Cat# ▲ ▼ | Product Name ▲ ▼ | Unit Size ▲ ▼ | Ex ▲ ▼ | Em ▲ ▼ |
36373 | Screen Quest™ Fluorimetric ELISA cAMP Assay Kit | 1 plate | 571 | 585 |
36374 | Screen Quest™ Fluorimetric ELISA cAMP Assay Kit | 10 plates | 571 | 585 |
Table 5. cAMP Conjugates and Reagents
Cat# ▲ ▼ | Product Name ▲ ▼ | Unit Size ▲ ▼ |
17102 | cAMP-NHS ester | 1 mg |
References
- “Camp Signaling Pathway.” Cusabio Life Science-Your Biology Science Partner, https://www.cusabio.com/pathway/cAMP-signaling-pathway-2.html.
- Zaccolo, Manuela, et al. “Subcellular Organization of the Camp Signaling Pathway.” Pharmacological Reviews, vol. 73, no. 1, 2020, pp. 278-309., https://doi.org/10.1124/pharmrev.120.000086.
- Sassone-Corsi, P. “The Cyclic AMP Pathway.” Cold Spring Harbor Perspectives in Biology, vol. 4, no. 12, 2012, https://doi.org/10.1101/cshperspect.a011148.
- Epstein, Paul M. “Different Phosphodiesterases (Pdes) Regulate Distinct Phosphoproteomes during Camp Signaling.” Proceedings of the National Academy of Sciences, vol. 114, no. 30, 2017, pp. 7741-7743., https://doi.org/10.1073/pnas.1709073114.
- Raker, Verena Katharina, et al. “The Camp Pathway as Therapeutic Target in Autoimmune and Inflammatory Diseases.” Frontiers in Immunology, vol. 7, 2016, https://doi.org/10.3389/fimmu.2016.00123.
- Milliken, Brandon, et al. “FRET Reporter Assays for Camp and Calcium in a 96-Well Format Using Genetically Encoded Biosensors Expressed in Living Cells.” BIO-PROTOCOL, vol. 10, no. 11, 5 June 2020, https://doi.org/10.21769/bioprotoc.3641.