Bridge PCR
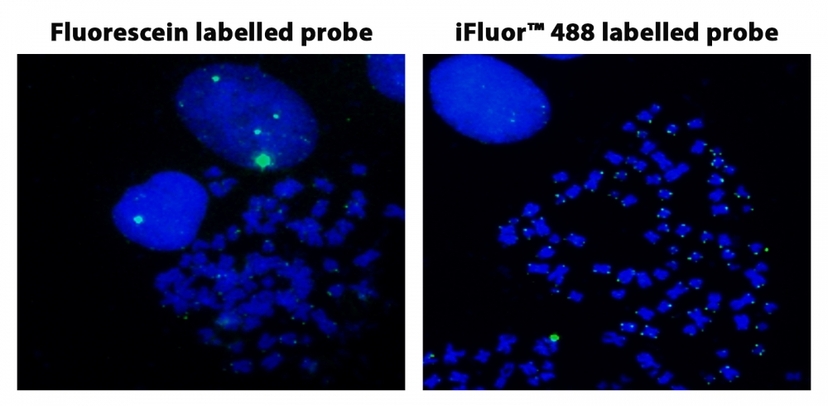
Fluorescence In Situ Hybridization of Fluorescein and iFluor® 488-dUTP labeled telomere probes in metaphase HeLa cells.
Outside of general PCR reagents like template DNA, DNA polymerase, and primers, there are two other important components that make bridge PCR unique:
- First, is the flow cell which is a solid support made of multiple channels. The flow cell adsorbs mobile DNA fragments and immobilizes the DNA within its channels. The DNA becomes bound to the flow cell in a manner that facilitates access to reactive enzymes, while simultaneously minimizing non-specific binding. Because the flow cell has multiple channels, different DNA constructs can also be analyzed at once.
- The second component includes adaptors which are known synthetic oligonucleotides sequences that become ligated to both and/or either ends of complementary DNA (cDNA) fragments.
How Bridge PCR Works
.png&w=1080&q=100)
Basic steps of Illumina next generation sequencing, showing how bridge amplification is used to multiply the clusters of fragmented template DNA. Figure made in BioRender.
The first step of bridge PCR is to prepare the flow cell. The flow cell glass is chemically derivatized, and then primers (complementary to the adapters) are complexed to the flow cell surface. Next, DNA must be fragmented into workable sized templates. Many methods of fragmentation can be used, including sonication, nebulization, and enzyme digestion, though it is important to choose a method that will deliver relatively small fragments, of generally the same size (e.g, between 200-500 bp). Next, adapters are ligated to the DNA fragments. Then, the DNA is denatured into ssDNA via heating the flow cell to around 95 ℃. After, ssDNA-adaptor constructs will anneal to complementary primer sites on the flow cell. Any DNA that is unbound to the flow cell is then denatured and washed away, leaving only target ssDNA available for subsequent amplification.
It is important to mention that the flow cell is full of a dense lawn of complementary oligos to the adaptor, to which all unbound ends of the ssDNA are attracted to. This attraction will force the ssDNA to bend over, forming a bridge-like shape, and attach its free end to another priming site on the flow cell. Then, sequencing reagents are added to the reaction, including dNTPs, primers, buffers, and DNA polymerase. DNA polymerase will use the bridged ssDNA as a template to attach and extend complimentary free dNTPs along the DNA. After the DNA polymerase extends the full length of the ssDNA it reaches the oligo attached to the flow cell. Then the flow cell is subject to denaturation once more which separates the DNA into two single strands that contain the same template but opposing primer constructs. All in all, steps can be repeated as necessary until the desired concentration of target DNA is reached.
Since the bridging occurrence of the ssDNA is localized, the end flow cell will have 'clusters' of identical DNA molecules. Each cluster contains ~1,000 copies of DNA and has a diameter on the flow cell of around 1 µm. These bound clusters can be visualized via fluorescent terminator nucleotides. In practice, after each cycle of amplification, primers and fluorescently labeled terminators are added to the flow cell. These terminators are versions of each nucleotide base that bring DNA synthesis to a halt. DNA polymerase then binds to the primer and adds a single fluorescently labeled terminator to the new DNA strand. As lasers are passed over the flow cell, the fluorescent terminators become activated. As each terminator base (A, C, T and G) gives off a different color, quantitative DNA sequencing analysis can be performed after each cycle of amplification. After the flow cell fluorescence is measured, fluorophores are cleaved, the flow cell is washed, and terminating bases are activated to enable another round of amplification.
Resources: |
Considerations of Bridge PCR
It should be noted that alone bridge PCR is not the most efficient method of clonal amplification. For example, around 35 amplification cycles may yield only ~1,000 copies of the amplicon. Bridge PCR is a relatively slow process and can only produce short reads between 30-35 bases long. Another con of the technique is that there is a high chance that the DNA template strands may hybridize with each other, rather than the anticipated primer, and create off-target products. In general, bridge PCR also has stricter requirements on the size of DNA that can be amplified. For these reasons, bridge PCR technologies have evolved significantly over the last few years.
Newer technologies offer cost-effective, high throughput, and easily scalable capabilities. Improvements and optimization of bridge PCR steps have also increased the accuracy and efficiency of the technique. Due to the versatility and flexibility of the technique, bridge PCR has a broad range of applications in genomics, transcriptomics, and epigenomics.
Product Ordering Information
Table 1. Deoxynucleotides (dNTPs) for use in PCR, real-time PCR, and reverse transcription PCR
Table 2. Sanger sequencing terminator kit and dye-labeled ddNTPs suitable for Sanger sequencing
Product ▲ ▼ | Ex (nm) ▲ ▼ | Em (nm) ▲ ▼ | Unit Size ▲ ▼ | Cat No. ▲ ▼ |
MagaDye™ 4 Color Sanger Sequencing Terminator Kit | 498 | 536/561/588/614 | 5 nmoles | 17068 |
MagaDye™ 535-ddGTP | 503 | 536 | 5 nmoles | 17063 |
MagaDye™ 535-ddGTP | 503 | 536 | 50 nmoles | 17067 |
MagaDye™ 561-ddATP | 498 | 561 | 5 nmoles | 17062 |
MagaDye™ 561-ddATP | 498 | 561 | 50 nmoles | 17066 |
MagaDye™ 588-ddTTP | 498 | 588 | 5 nmoles | 17061 |
MagaDye™ 588-ddTTP | 498 | 588 | 50 nmoles | 17065 |
MagaDye™ 613-ddCTP | 498 | 614 | 5 nmoles | 17060 |
MagaDye™ 613-ddCTP | 498 | 614 | 50 nmoles | 17604 |